Viscosity (resin) - A203
Viscosity (resin) | |
---|---|
Foundational knowledge article | |
Document Type | Article |
Document Identifier | 203 |
Themes | |
Relevant Class |
Material |
Tags | |
Prerequisites |
Introduction[edit | edit source]
The viscosity (\(\mu\)) of a fluid represents its resistance to deformation at a given rate. The lower the viscosity, the more easily resin flows. Resin viscosity ranges considerably across chemistries and formulations. By scientific definition, viscosity is a measure of a material’s resistance to deformation. For liquids, it is in response to imposed shear stresses.
Scope[edit | edit source]
This page defines resin viscosity and explains its significance to composites processing. Typical values for various polymer matrices and everyday encountered fluids are provided. Finally, a brief introduction to viscosity measurement is provided.
Significance[edit | edit source]
In composites processing, viscosity is an indicator of how easily the resin matrix will mix with the reinforcement and how well it will stay in place during processing.
Resin viscosity is significant for:
- Fibre wetout
- Controlling how well/long the resin holds in place (e.g. critical in adhesives, control of resin bleed, laminate gas pathways for laminate gas evacuation, etc.)
Prerequisites[edit | edit source]
Recommended documents to review before, or in parallel with this document:
Definition[edit | edit source]
The viscosity (\(\mu\)) of a fluid represents its resistance to deformation at a given rate, and in simplest form is:
Where,
\(\tau = \) Shear stress
\(\dot{\gamma} = \) Shear rate
Whereas the viscosity of a thermoplastic drops exponentially but monotonically with increasing temperature, thermoset resins behave in a more complex fashion. Initially, viscosity drops in the same manner as a thermoplastic, but once cure kicks off, the increasingly higher molecular weight and cross-linked polymer structure increases the viscosity rapidly, until gelation occurs, and viscosity becomes unmeasurably large. Past gelation, the material is no longer a fluid and should be treated as a viscoelastic solid.
Typical Property Values[edit | edit source]
The following table, reproduced from Hoa [1], shows some typical values of various polymer matrix materials, and other common fluids encountered in everyday life.
Material | 20 °C | T °C |
---|---|---|
(mPa·s) | (mPa·s) | |
Air | 0.0187 | |
Water | 1 | |
Polyester (thermoset) | 100-300 | |
Vinyl ester (thermoset) | 100-300 | |
#10 Motor oil | 500 | |
Golden syrup | 2500 | |
Epoxy (Shell Epon 828 14phr MPDA, 15 phr BGE) (thermoset) | 600 | |
Epoxy (Shell 826 16 phr MPDA, 10 phr BGE) (thermoset) | 750 | |
Epoxy (Dow 332 16phr MPDA, 10 phr BGE) (thermoset) | 500 | |
Molasses | 105 @ 25 °C | |
Epoxy 5208 (thermoset) | 100 @ 177 °C | |
BMI (thermoset) | 1000 @ 150 °C | |
Ryton (thermoplastic) | 107 @ 313 °C | |
PEEK (thermoplastic) | 106 @ 400 °C | |
Ultem (thermoplastic) | 108 @ 305 °C | |
Torlon (thermoplastic) | 109 @ 350 °C |
The viscosity of thermoplastics is orders of magnitude greater than the short monomers that make up thermosetting polymers prior to the thermoset curing process. Thermoplastics require elevated processing temperature in order to flow. Even heated, they still require substantial applied pressure to accommodate their relatively high viscosity.
Process Dependencies[edit | edit source]
Resin viscosity ranges considerably across different resin chemistries and formulations. Additionally, resin viscosity is directly influenced by the following factors:
- Temperature (increase temperature = viscosity decrease)
- Degree of cure – thermoset polymers (increase DOC = viscosity increase)
- Resin fillers and tougheners (increase particulate content = viscosity increase)
Thermal management of viscosity[edit | edit source]
Two of the above listed viscosity influencing factors can be controlled during processing through thermal management – control of temperature. The effects of temperature and its downstream linked degree of cure (DOC) are illustrated below for a thermoset resin system.
Resin viscosity drops as a function of increasing temperature. Countering this resin viscosity drop, the thermoset crosslinking reaction takes place with elevated temperature and sees increasing molecular weight and restriction in mobility of the polymer – resulting in a corresponding increase in resin viscosity as the thermosetting reaction progresses. During a heated cure cycle, the resin therefore experiences the following sequence:
- An initial lowering of resin viscosity as the resin is being heated, this is quickly countered and overtaken with,
- An increasing resin viscosity due to the thermoset reaction effects.
- Resin viscosity will continue to increase until the resin reaches the gelation point, where it takes on the behaviour of a semi-solid.
Viscosity relationship to temperature and degree of cure[edit | edit source]
The resin viscosity relationship to temperature and its degree of cure can be described using an empirical model for an Arrhenius-type equation shown below:
\(\mu = Ae^{\frac{\Delta E}{RT}f(x)}\)
Where,
\(A = \) A constant
\(\Delta E = \) Activation energy
\(RT = \) Kinetic energy as a function of temperature
\(f(x) = \) Dependency on the degree of cure
These two competing factors (temperature, and DOC – resin reaction) affecting resin viscosity must be balanced for setting the resin’s processing window. This is of particular importance in resin infusion process steps, where liquid resin must fully infuse the fibre preform – sometimes with flow lengths in the range of metres. Increasing temperature lowers the resin viscosity which aids in resin/fibre wetout. This is the fundamental motive behind the practice of performing heated resin infusion. However, in doing so, there is also an associated risk that the elevated temperature kicks off and accelerates the resin reaction. This can quickly become the dominating effect by rapidly and irreversibly increasing the resin viscosity. If the resin’s degree of cure progresses too quickly, this will quickly inhibit the resin’s ability to flow and ultimately reduce the time available for the resin to flow. Insufficient resin infusion may occur, and result in dry spots with a partially resin infused fibre preform.
Resin fillers and tougheners[edit | edit source]
Whether as resin fillers or matrix toughers, the addition of solid particles to a liquid resin results in a viscosity increase to the combined mix. At low particle concentrations, and for spherical particles greater than 1 \(\mu\)m in size, this relationship is described Einstein’s equation that can predict the effective resin viscosity of the mix.
Einstein's equation for dilute suspensions:
\(\mu_{eff} = \mu_0 (1 + 2.5 \phi)\)
Where,
\(\mu_{eff} = \) Resulting effective mix viscosity
\(\mu_0 = \) Viscosity with no particle content
\(\phi = \) Volume fraction of particles
The viscosity increase is more prevelent for higher particle concentrations and smaller particle sizes, where particle-to-particle interactions result in even greater viscosity increases than the relationship predicted by Einstein’s equation. This viscosity increase needs to be considered for infusion processes where high particle loading is desired, or when small particles such as nano-scale particle tougheners are intended to be added to the liquid resin.
The following knowledge base article by Anton Paar (equipment manufacturers of rheometers) discusses the influences of particles on suspension rheology [2].
Composite Viscosity[edit | edit source]
Link to Link to main Viscosity (composite) page
While it is common practice to isolate and examine the viscosity of the resin matrix component on its own, it is important to consider the influence of the other composite constituent materials when the entire combined system of the matrix and the reinforcement materials are considered together as a whole viscous material.
When examining the composite material system as a whole, the addition of the reinforcement fibres has an enormous influence viscoelastic response of the material system. The fibres are able to resist shear deformation in proportion to the shear modulus of the fibre bed due to the waviness of the fibres, with this effect being higher for high volume fraction composites [3]. The fibre bed’s ability to absorb and dissipate the applied shear forces acts to essentially “thicken” the viscous behavioural response of the fibre/resin composite material. As a result, the effective viscosity of the composite material is much higher when compared to examining solely the resin viscosity on its own.
Click here to visit the Viscosity (composite) page and learn more.
Measurement[edit | edit source]
Resin viscosity is typically measured using a rheometer with parallel plate geometry, and disposable aluminum plates are used since the material cures to the plates. A rheometer is a specialized laboratory device that examines the response of a liquid, suspension, or soft viscous solid when subjected to applied forces. In particular, the method described here concerns the use of rotational shear type rheometers, examining a liquid’s response to shear forces.
Viscosity measurements can be made using two different plate rotational motions:
- Continuous rotation
- Oscillation
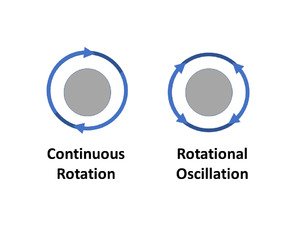
Rheometers can operate with either continuous rotation or rotational oscillation motion of the plate, cone, etc. setup. The information that can be obtained differs between the two motions, and each has its advantages and disadvantages.
Continuous Rotational Motion | Oscillating Rotational Motion |
---|---|
Advantages:
|
Advantages:
|
Limitations:
|
Limitations:
|
To approximate the resin’s gelation point, both rheometer motions can be used. However, for curing polymers, oscillating motion is generally considered preferable as it can measure and provide information past the gelation point until the sample becomes a solid [5].
To learn more about viscosity and viscosity measurement, the following rheology knowledge base document by Anton Paar (manufacturer of rheometer equipment) [4] is a good resource.
Related pages
References
- ↑ [Ref] Hoa, S V (2018). Principles of the Manufacturing of Composite Materials. DEStech Publications, Incorporated. ISBN 9781605954219.CS1 maint: uses authors parameter (link) CS1 maint: date and year (link)
- ↑ [Ref] Anton Paar. "The influence of particles on suspension rheology". Retrieved 24 March 2021.CS1 maint: uses authors parameter (link)
- ↑ [Ref] Thorpe, Ryan J. (2013). Experimental Characterization of the Viscoelastic Behavior of a Curing Epoxy Matrix Composite from Pre-Gelation to Full Cure. MASc thesis (Thesis). doi:10.14288/1.0073820.CS1 maint: uses authors parameter (link)
- ↑ 4.0 4.1 4.2 [Ref] Anton Paar. "Basics of rheology". Retrieved 21 January 2021.CS1 maint: uses authors parameter (link)
- ↑ [Ref] Anton Paar. "Time-dependent behavior with gel formation or curing". Retrieved 21 January 2021.CS1 maint: uses authors parameter (link)
About | Help |

Welcome
Welcome to the CKN Knowledge in Practice Centre (KPC). The KPC is a resource for learning and applying scientific knowledge to the practice of composites manufacturing. As you navigate around the KPC, refer back to the information on this right-hand pane as a resource for understanding the intricacies of composites processing and why the KPC is laid out in the way that it is. The following video explains the KPC approach:
Understanding Composites Processing
The Knowledge in Practice Centre (KPC) is centered around a structured method of thinking about composite material manufacturing. From the top down, the heirarchy consists of:
- The factory
- Factory cells and/or the factory layout
- Process steps (embodied in the factory process flow) consisting of:
The way that the material, shape, tooling & consumables and equipment (abbreviated as MSTE) interact with each other during a process step is critical to the outcome of the manufacturing step, and ultimately critical to the quality of the finished part. The interactions between MSTE during a process step can be numerous and complex, but the Knowledge in Practice Centre aims to make you aware of these interactions, understand how one parameter affects another, and understand how to analyze the problem using a systems based approach. Using this approach, the factory can then be developed with a complete understanding and control of all interactions.
Interrelationship of Function, Shape, Material & Process
Design for manufacturing is critical to ensuring the producibility of a part. Trouble arises when it is considered too late or not at all in the design process. Conversely, process design (controlling the interactions between shape, material, tooling & consumables and equipment to achieve a desired outcome) must always consider the shape and material of the part. Ashby has developed and popularized the approach linking design (function) to the choice of material and shape, which influence the process selected and vice versa, as shown below:
Within the Knowledge in Practice Centre the same methodology is applied but the process is more fully defined by also explicitly calling out the equipment and tooling & consumables. Note that in common usage, a process which consists of many steps can be arbitrarily defined by just one step, e.g. "spray-up". Though convenient, this can be misleading.
Workflows
The KPC's Practice and Case Study volumes consist of three types of workflows:
- Development - Analyzing the interactions between MSTE in the process steps to make decisions on processing parameters and understanding how the process steps and factory cells fit within the factory.
- Troubleshooting - Guiding you to possible causes of processing issues affecting either cost, rate or quality and directing you to the most appropriate development workflow to improve the process
- Optimization - An expansion on the development workflows where a larger number of options are considered to achieve the best mixture of cost, rate & quality for your application.