Convection - A106
Convection | |
---|---|
Foundational knowledge article | |
![]() | |
Document Type | Article |
Document Identifier | 106 |
Themes | |
Relevant Class |
Material |
Tags | |
Prerequisites |
Introduction[edit | edit source]
Convective heat transfer, known as convection, is the heat transport mechanism associated with the flow of fluids acting as the carrier of thermal energy. It involves a combination of the sub-mechanisms of conduction and advection (bulk fluid motion). A classic physics example of convection is the heat transfer that occurs between a solid surface of a plate and fluid flowing over its surface. Locally, heat transfer occurs by conduction at the interface between the surface and the fluid, and away from the surface within the moving fluid [1]. Together, this combined process forms the convective heat transfer process [2].
Scope[edit | edit source]
This page explains the basics of thermal convection and puts the heat transfer mechanism in the context of composites processing.The difference between forced convection and natural convection is explained and the convective heat transfer coefficient (HTC), used to quantify this heat transfer mecahnism, is defined. Typical HTC values encountered in composite processes with factors that influence it are also given.
Significance[edit | edit source]
Convection is the heat transfer mechanism between fluid (or gas) in contact with a solid surface. In composites processing, convection is an important heat transfer mechanism occurring in oven and autoclave processing methods. In these processes, heat is transferred between the hot gas environment to the composite part and tooling. This is also true for room temperature processing methods where convective heat transfer is taking place between the curing part/tool and the ambient air (gas) environment of the room.
Prerequisites[edit | edit source]
Recommended documents to review before, or in parallel with this document:
Convection Overview[edit | edit source]
Heat transfer by convection between a fluid and a solid surface is expressed by Newton’s law of cooling [3][2] (the same expression applies for heating):
\(q = h\, \Delta T\) Newton’s law of cooling, where:<br />\(q\) = Heat flux [W/m<sup>2</sup>],<br />\(h\) = Convective heat transfer coefficient (HTC) [W/m<sup>2</sup>·K], and<br />\(\Delta T\) = Temperature difference between fluid and solid surface [K],<br />
The above expression is sometimes expressed as a heat rate – where surface area is explicitly part of the expression:
\(\dot{Q} = h\, A\, \Delta T\) Heat rate due to convection, where:<br />\(\dot{Q}\) = Heat rate [W],<br />\(h\) = Convective heat transfer coefficient (HTC) [W/m<sup>2</sup>·K],<br />\(A\) = Surface area of solid surface [m<sup>2</sup>], and<br />\(\Delta T\) = Temperature difference between fluid and solid surface [K],<br />
A key parameter in the above expressions is the convective heat transfer coefficient (HTC). The HTC depends on the fluid-flow conditions, thermophysical properties of the fluid, and flow path [2].
The HTC value quantifies the rate of heat transfer from the solid surface to the fluid (through the fluid film boundary layer). The greater the HTC value, the higher the heat transfer between the fluid and solid body surface.
Mechanisms[edit | edit source]
A further differentiation of convective heat transfer is made between two of the possible fluid flow conditions:
- Forced convection – where fluid flow is caused by some external means (e.g. a blowing fan), and
- Natural convection – where fluid motion occurs naturally from the localized spatial temperature gradient inducing localized density changes within the fluid and a subsequent fluid motion.
Forced convection[edit | edit source]
Forced convection implies a mechanically induced fluid flow and is the scenario encountered fluid motion is caused by external means such as fan, blower, pump or wind [2], causing an external pressure difference in the fluid [1]. As shown in the earlier table, forced convection results in a much higher convective heat transfer coefficient (HTC) value and improved heat transfer. Simply put, the forced fluid flow improves the heat transfer process between the fluid and the solid surface.
Examples of forced convection situations in composites processing are fan blown ovens (convection ovens) and autoclave heating equipment. The blown gas over the curing part is beneficial, improving the heating and cooling processes.
Natural convection[edit | edit source]
In natural or free convection, the fluid motion is purely from gravity and the natural fluid circulation due to the hot and cold fluid mixing. Fluid close to the heat source reduces in density and rises, and is subsequently replaced with surrounding colder and denser fluid. The resulting convective heat transfer coefficient (HTC) in natural convection scenarios is much lower than the HTC in forced convection. As a result, the heat transfer ability between the fluid and solid surface is reduced when compared to the mechanically induced forced convection scenario.
In composites processing, natural convection is encountered with an oven without a blown fan (natural convection oven), and room temperature curing where the gas motion of the ambient environment is occurring naturally.
Effect on heat transfer coefficient[edit | edit source]
The effects of fluid flow on convective heat transfer is shown in the example HTC ranges provided below for different gasflow conditions, representing natural convection and forced convection conditions (source: [2]).
Gasflow Condition | HTC
[W/m2·K] |
HTC
[BTU/ft2·hr·°F] |
---|---|---|
Stagnant Gas, Natural Convection | 5-25 | 1-4 |
Forced Convection, air/gas (1 atm) | 10-250 | 2-44 |
While the above table of values show that a HTC as high as 250 W/m2·K can be obtained with forced convection gasflow (at 1 atm pressure), the expected HTC in composites processing are typically lower. This is discussed in the next section.
Convective Heat Transfer in Composites Processing[edit | edit source]
Convective heat transfer is dependent on any factor influencing the properties of the heat transfer fluid and its flow path. In composites processes using oven or autoclave curing where hot gas (air or nitrogen) is the fluid, these factors include:
- Gas velocity
- Gas pressure
- Gas temperature
- Laminar or turbulent flow
- Surface orientation
- Surface geometry
Effect of: gas velocity, pressure, and temperature[edit | edit source]
The first of the 3 factors on the above list (gas velocity, gas pressure, gas temperature) are regulated by the heating equipment. Their influence on convective heat transfer will be briefly discussed here.
With the capability limits of the heating equipment, and the material manufacturer defined curing conditions for the matrix; the influencing factors of imposed gas velocity, pressure and temperature fall within a limited range for composites processing. As a result, HTC values under 200 W/m2·K are typically observed for autoclave processing, and a lower HTC is observed for oven processing. In the following figure, the effects of gas velocity, pressure, and temperature on the convective heat transfer coefficient (HTC) within the bounds that can be achieved in autoclave processing are shown.
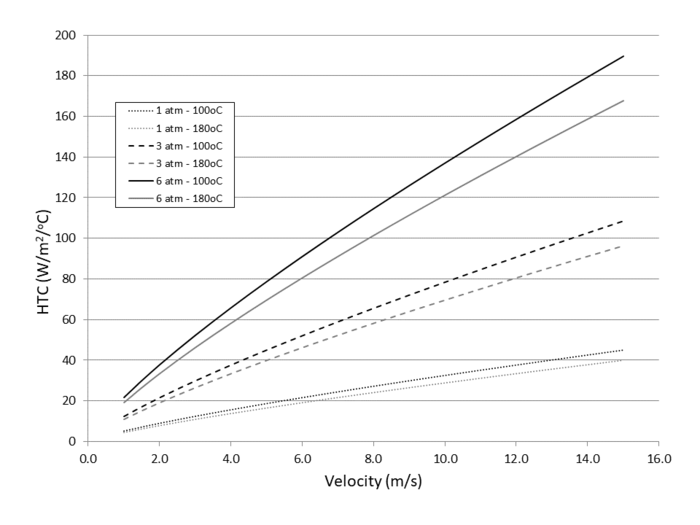
The curves at 1 atm pressure can be considered the behaviour obtained in a forced convection oven. While the applied pressure results of 3 atm and 6 atm represent the applied pressure that can provided by an autoclave. Depending on the applied process conditions in the autoclave, the convective heat transfer HTC varies, where it can range from as low as 10 W/m2·K to nearly as high as approaching 200 W/m2·K. At applied pressure conditions, a significant sensitivity to gas velocity is observed, where an increase in the gas velocity has a corresponding large influence on dramatically increasing the effective HTC.
As illustrated in the above example, the following effects can be summarized:
Factor | HTC Outcome | Notes: |
---|---|---|
Increasing Gas Velocity | Increasing Convective HTC |
|
Increasing Gas Pressure | Increasing Convective HTC |
|
Increasing Temperature | Decreasing Convective HTC |
|
As summarized in the above table, some factors that can be imposed like pressure or gas velocity can improve the convective heat transfer, while other necessary factors like increasing temperature that required for matrix curing, decreases the convective heat transfer effect. For autoclave processing, a simple relationship was observed for turbulent gas flow by Johnston et al. [5].
\(h \varpropto \left ( \frac{P}{T} \right )^{\frac{4}{5}}\) Relationship between pressure, temperature and convective heat transfer coefficient for turbulent flow:<br />\(h\) = Convective heat transfer coefficient (HTC),<br />\(P\) = Pressure, and<br />\(T\) = Temperature
Effect of: surface orientation and surface geometry[edit | edit source]
Influence of tooling shape on airflow
Influence of part shape on airflow
The surface orientation and geometry of the tool-part assembly may play a significant role in blocking or redirecting gas flow in a convective heating environment. In turn, this may alter the boundary layer across the tool/part, thereby altering the laminar and turbulent flow regions, and thus the HTC. The impact the tool and part(s) have on flow are heavily dependent on the their placement within the heating environment. To learn more about how the tool/part may influence gas flow, click on the links above.
Related pages
References
- ↑ 1.0 1.1 [Ref] Böckh, Peter von; Wetzel, Thomas. Heat transfer: basics and practice. Springer. ISBN 9783642191824.CS1 maint: uses authors parameter (link)
- ↑ 2.0 2.1 2.2 2.3 2.4 [Ref] Karwa, Rajendra et al. (2020). Heat and Mass Transfer. Springer Singapore. ISBN 9811539871.CS1 maint: extra punctuation (link) CS1 maint: uses authors parameter (link) CS1 maint: date and year (link)
- ↑ [Ref] Bejan, Adrian (2013). Convection heat transfer, fourth edition. Wiley. ISBN 9780470900376.CS1 maint: uses authors parameter (link) CS1 maint: date and year (link)
- ↑ [Ref] Fernlund, Göran et al. (2018). Talreja, Ramesh (ed.). Autoclave Processing. Elsevier Science Ltd. doi:10.1016/B978-0-12-803581-8.09899-4. ISBN 9780081005347.CS1 maint: extra punctuation (link) CS1 maint: uses authors parameter (link) CS1 maint: date and year (link)
- ↑ [Ref] Johnston, Andrew et al. (1998). An Investigation of Autoclave Convective Heat Transfer.CS1 maint: extra punctuation (link) CS1 maint: uses authors parameter (link) CS1 maint: date and year (link)
About | Help |

Welcome
Welcome to the CKN Knowledge in Practice Centre (KPC). The KPC is a resource for learning and applying scientific knowledge to the practice of composites manufacturing. As you navigate around the KPC, refer back to the information on this right-hand pane as a resource for understanding the intricacies of composites processing and why the KPC is laid out in the way that it is. The following video explains the KPC approach:
Understanding Composites Processing
The Knowledge in Practice Centre (KPC) is centered around a structured method of thinking about composite material manufacturing. From the top down, the heirarchy consists of:
- The factory
- Factory cells and/or the factory layout
- Process steps (embodied in the factory process flow) consisting of:
The way that the material, shape, tooling & consumables and equipment (abbreviated as MSTE) interact with each other during a process step is critical to the outcome of the manufacturing step, and ultimately critical to the quality of the finished part. The interactions between MSTE during a process step can be numerous and complex, but the Knowledge in Practice Centre aims to make you aware of these interactions, understand how one parameter affects another, and understand how to analyze the problem using a systems based approach. Using this approach, the factory can then be developed with a complete understanding and control of all interactions.
Interrelationship of Function, Shape, Material & Process
Design for manufacturing is critical to ensuring the producibility of a part. Trouble arises when it is considered too late or not at all in the design process. Conversely, process design (controlling the interactions between shape, material, tooling & consumables and equipment to achieve a desired outcome) must always consider the shape and material of the part. Ashby has developed and popularized the approach linking design (function) to the choice of material and shape, which influence the process selected and vice versa, as shown below:
Within the Knowledge in Practice Centre the same methodology is applied but the process is more fully defined by also explicitly calling out the equipment and tooling & consumables. Note that in common usage, a process which consists of many steps can be arbitrarily defined by just one step, e.g. "spray-up". Though convenient, this can be misleading.
Workflows
The KPC's Practice and Case Study volumes consist of three types of workflows:
- Development - Analyzing the interactions between MSTE in the process steps to make decisions on processing parameters and understanding how the process steps and factory cells fit within the factory.
- Troubleshooting - Guiding you to possible causes of processing issues affecting either cost, rate or quality and directing you to the most appropriate development workflow to improve the process
- Optimization - An expansion on the development workflows where a larger number of options are considered to achieve the best mixture of cost, rate & quality for your application.